Specific heat capacity
Another effect of hydrogen bonding between water molecules is that it takes a lot of energy (supplied as heat) to boil or evaporate water, i.e., to break the bonds between the water molecules. Water is therefore described as having a high specific heat capacity, where the specific heat capacity is a measure of how much energy it takes to raise the temperature of 1 kg of a particular substance by 1 °C. This makes water fairly stable over a wide temperature range and it helps to protect cells, which contain water, even if external temperatures are high.
Water’s specific heat capacity also allows large bodies of water, such as oceans, to absorb a lot of heat. Conversely, landmasses have low specific heat capacity and heat up more than oceans. Oceans can absorb the heat of nearby landmasses, moderating the temperature and - on a planetary scale - the climate. This allows ecosystems to thrive that would otherwise suffer from large temperature fluctuations. Although it takes longer for water to heat up than other substances, when water evaporates, the cooling effect is efficient. This is why forests are cooler in the summer (from the evaporation of water from the leaves) and why we sweat when our bodies need to cool down.
The transition of water between liquid, gas and solid (ice) is not only dependent on temperature, but also pressure – in particular the pressure of the atmosphere. Figure 10 shows which phase of water might exist at any given temperature and pressure.

-
Look at Figure 10 and refer back to Table 1 in Section 1. Considering the average temperature and pressure on Mars, do you expect water to be solid, liquid or gas?
-
The average temperature on Mars is -63 °C and the average pressure is 75 mbar. Reading from Figure 10, this would suggest that any water on Mars would be ice (a solid).
The likely presence of ice on Mars is still significant. When water freezes, its molecular structure becomes ‘frozen’ into a uniform, three-dimensional arrangement. Figure 11 shows that this structure has a repeating pattern, characterised by large hexagonal open channels. This structure means the molecules are more spread out than in liquid water, so ice is less dense than liquid water and can float. This is very important for life on Earth because it protects the water below the ice from cold air temperatures. Water under the ice stays liquid, providing life a more clement range of temperatures in which life can survive. As you will see later, ice on Mars may also protect bodies of liquid water that could be significant in the search for life.
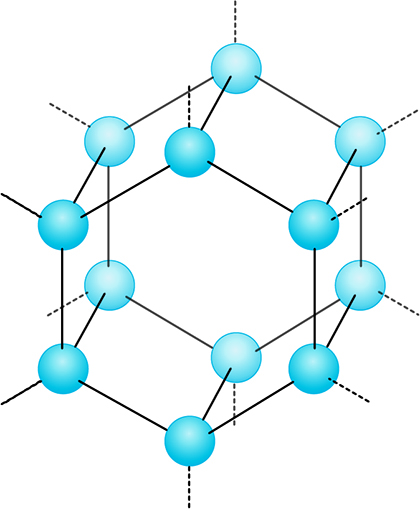
The ‘openness’ of the ice structure also has another important function - it can allow other molecules, such as methane, to become trapped. On Earth such systems - called clathrates - have been found underneath ocean floors. Methane is a molecule that can be generated by life (and also by natural processes), so finding these on Mars has been a focus of some exploration missions looking for evidence that life was once present.