Production of FPH from aquaculture waste
The production of hydrolysates from aquaculture fish by-products (heads, frames, trimmings, etc.) involves a set of sequential stages that transforms these substrates into a liquid rich in proteins and peptides that can be subsequently dried to produce a powder if the application of the FPH requires it (Figure 4).
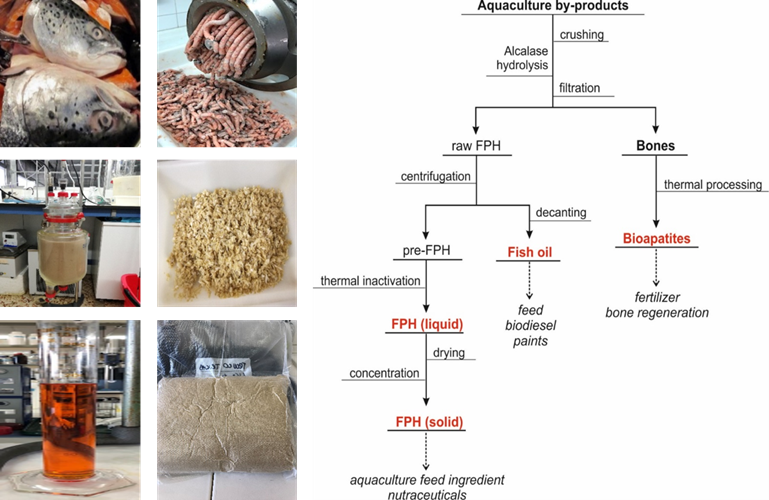
Figure 4. Pictures showing the different products generated in the enzyme hydrolysis of salmon heads (left). Flowchart dealing with the production of FPH from aquaculture wastes together with the recovery of fish oil and bioapatites (right).
The first step is to homogenize the waste using a mincer, grinder, or crusher. This grinding material is then hydrolysed under the conditions exposed in Table 1, and which were optimised according to the experimental and mathematical methodology indicated in the previous section. From the ample number of commercial proteases present in the market, alcalase is one of the best enzymes to digest fish. It is a very versatile biocatalyst that has shown an excellent capacity and effectiveness for the hydrolysis of several marine substrates such as cartilages, heads, skeletons, and viscera.
The optimal values for alcalase proteolysis of fish farming wastes (heads, viscera, and trimmings + frames) from Atlantic salmon (Salmo salar), rainbow trout (Oncorhynchus mykiss), turbot (Scophthalmus maxima), seabream (Sparus aurata), and seabass (Dicentrarchus labrax), ranged from 8.2-9.0 for pH, 56-64ºC for temperature and 0.1-0.2% (v/w) for enzyme concentration.
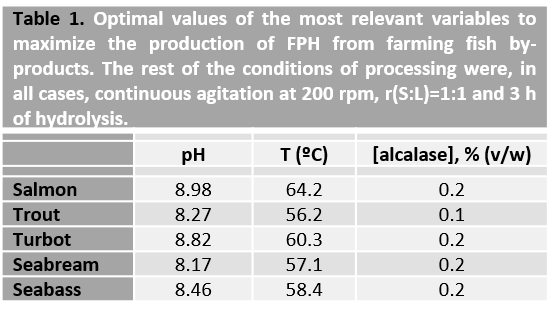
Once the time of hydrolysis has finished, the content of the pH-stat reactor is passed throughout a filter or sieve with a suitable pore size to separate non-enzymatically digested substrates, basically remains of clean bones, from the liquid raw-FPH. This liquid phase is then centrifuged at high speed (>14,000 x g for 30 min) and decanted to promote the separation of the fat fraction presents. The yield of oil recovered is dependent on the kind of species and tissue employed. Therefore, this step of fat separation can be omitted when low-fat fish are processed. This is not the case with aquaculture substrates in which the high proportion of oil forces its removal.
The pre-FPH free of most oil is quickly heat-treated (90-95°C for 10-15 min) in order to inactivate the protease and thus end the process of proteolysis. The chemical properties and mass balance of aquaculture FPH from head and trimmings + frames are shown in Table 2. A remarkable concentration of soluble protein was produced after the excellent proteolysis performance of alcalase (Ydig> 78%), together with the complete recovery of the bones and most of the oil. The quality of the protein in FPH was revealed by its high in vitro digestibility and a high proportion of essential amino acids present in these materials. The average molecular weight of hydrolysed proteins for all farming species was in the interval 1-2 kDa, indicating their potential to be incorporated into aquatic diets as substitutes for fish meal.
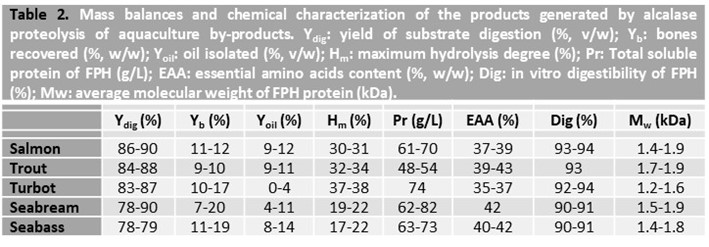
Although the hydrolysate obtained can be used in its liquid form for some applications (e.g., as fish attractant), the most habitual way for conservation and use is as a solid, for instance, when it is applied as an ingredient of aquaculture feed. Prior to drying, it is a commonly necessary step of concentration (using ultra- or nanofiltration membranes or by vacuum evaporation). This is because the most energy-demanding and time-consuming process is drying and, therefore, the lower the volume of FPH to be dehydrated, the lower the hydrolysate costs will be.
In order to produce a dry hydrolysate with a moisture content lower than 10% (w/w), the two most extended techniques in the industry are:
(i) Lyophilization (or freeze-drying), in which after freezing the sample and applying an intense vacuum in the drying chamber, the hydrolysate is dehydrated –ice removal– by means of a sublimation process.
(ii) Spray drying, in which the hydrolysate concentrated is atomized, dehydrated in the drying chamber by contact with a hot gas, and deposited as a powder in the separator cyclone by centrifugal forces.
Freeze-drying is a more expensive technology than spray-drying, but the recovery of solid hydrolysate is complete whereas a certain amount of solids are commonly lost in the spray drying treatment. However, a subsequent step of milling is likely needed for the FPH dried by lyophilization to obtain a fine powder. In both cases, to avoid microbial spoilage and oxidation, the final solid FPH is vacuum packed and stored at 4ºC until use. The production of aquaculture FPH at the pilot plant scale using the aforementioned scheme of operation was confirmed (Figure 5). Mass balance and chemical attributes of the hydrolysates were in agreement with those achieved at the 5L-reactor (Table 2). Those dry FPH demonstrated to be excellent substrates for their use as ingredients in the formulation of fish farming feed, presenting a proximal composition of (in %, dry basis): moisture lower than 9%, total protein higher than 70%, ash around 11-14% and total fat lower than 10%.
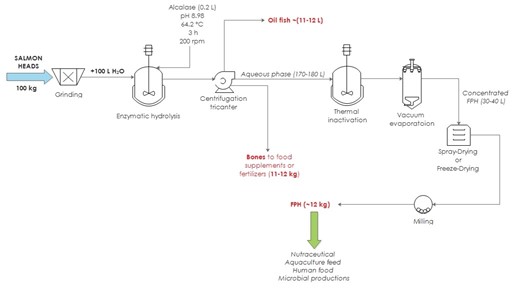
The two co-products recovered concomitantly to the production of FPH, fish oil, and the clean bones that are not digested by enzyme action, are also materials of potential interest in different fields of application (Figure 4). The composition of aquaculture oils was predominant in oleic, linoleic, and palmitic acids, with an omega-3/omega-6 ratio lower than 1, reducing its use for nutraceuticals but being interesting for biodiesel and paints production as well as feed formulations. Aquaculture bones, after thermal calcination, are an attractive source of biogenic calcium for nutraceutical and bone regeneration ingredients, showing a Ca/P ratio in the range of 1.66-1.77, similar to the bioapatites present in human bones.